This month’s maker is Changhua Mu, PhD, assistant professional researcher (II) in Professor Robert Flavell’s laboratory in the Department of Radiology and Biomedical Imaging at UCSF. Let’s take a look at what they made.
Q: What did you make?
We created an apparatus that produces a special compound called hyperpolarized (HP) 13C-bicarbonate, which helps with advanced magnetic resonance imaging (MRI) scans. These scans can detect a feature of aggressive tumors called “tumor acidosis,” assisting physicians to identify and classify prostate cancer in patients. This machine is part of ongoing clinical trials (NCT05851365).
To make the HP 13C-bicarbonate, we use a process called Dynamic Nuclear Polarization (DNP). The process involves breaking down one substance, HP 13C-glycerol carbonate, into another substance, HP 13C-carbonate, using a chemical reaction. Then, we adjusted the chemical balance with a buffer system to get the final HP 13C-bicarbonate. The apparatus is designed to handle this chemical process and then purify and sterilize the final product so it is safe to inject into patients.
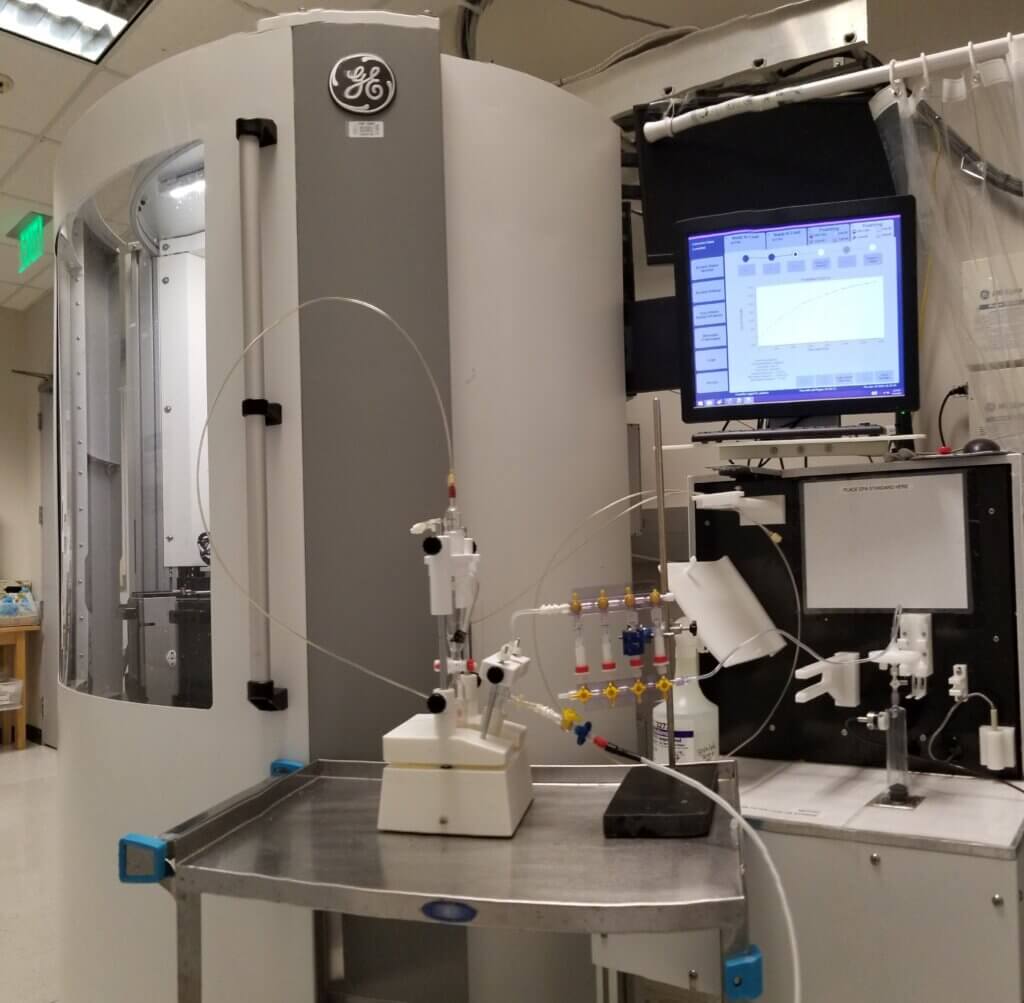
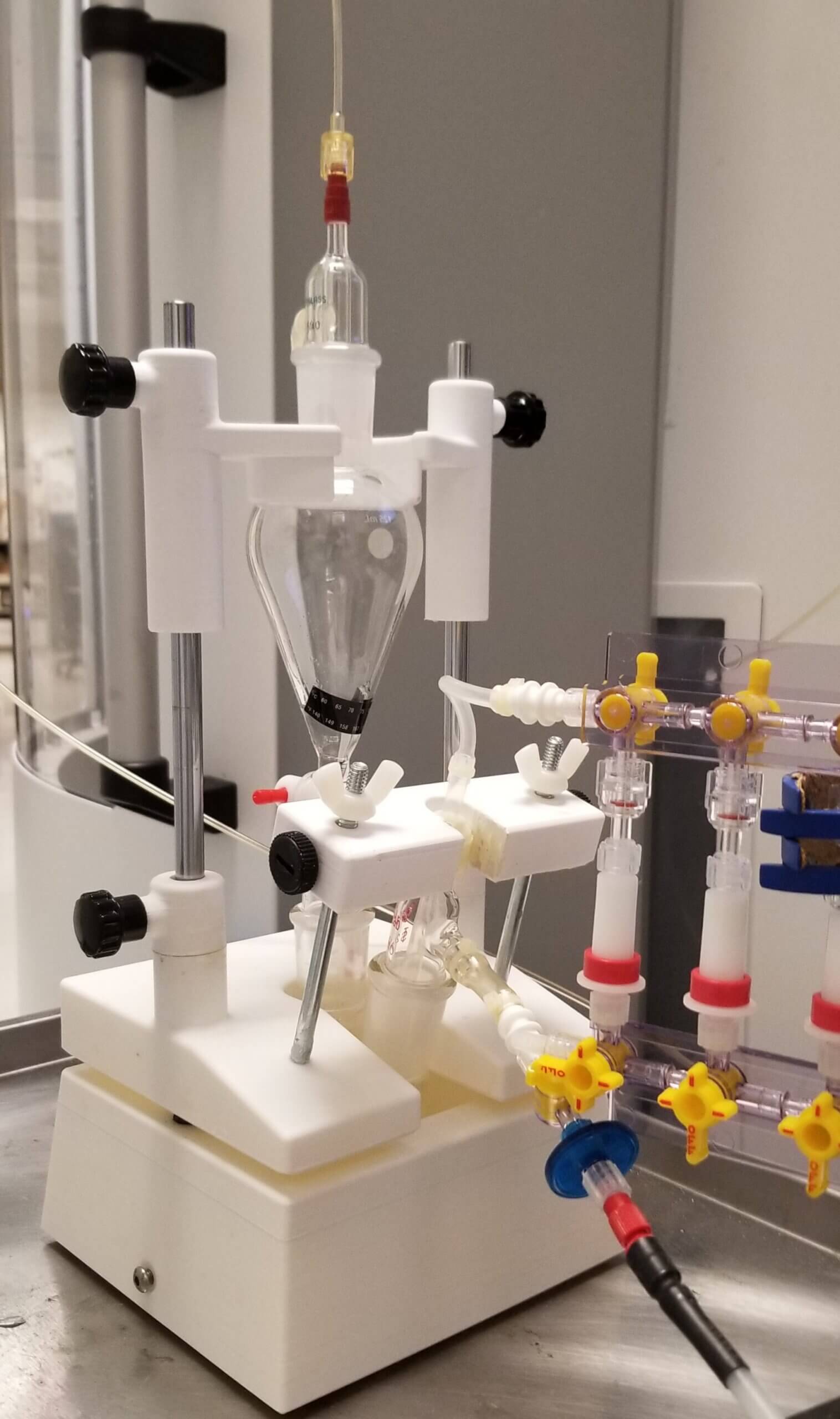
Q: Why did you want to make it?
We developed a new imaging method, HP 13C MRI (a special type of magnetic resonance imaging), to measure tissue pH (acidity or basicity) levels, which can help detect certain health conditions. We were granted Investigational New Drug (IND) approval from the U.S. Food and Drug Administration (FDA).
The device currently available for preparing clinically applicable hyperpolarized 13C contrast agent (a substance injected into the body to enhance the visualization of specific tissues or structures during the scan) is the 5T GE SPINlab. This clinical polarizer is only designed to work with well-established specific 13C agents, such as 13C pyruvic acid. Unfortunately, it cannot handle other chemicals we need for our new method, such as 13C-glycerol carbonate (13C-GLC).
To solve this problem, we built a custom apparatus that includes 3D-printed parts (specially made components), combined with linear bearings, guides, and additional hardware. The design of this machine can handle the unique chemical reactions and processes required to create the materials for our imaging method.
Q: What was your process?
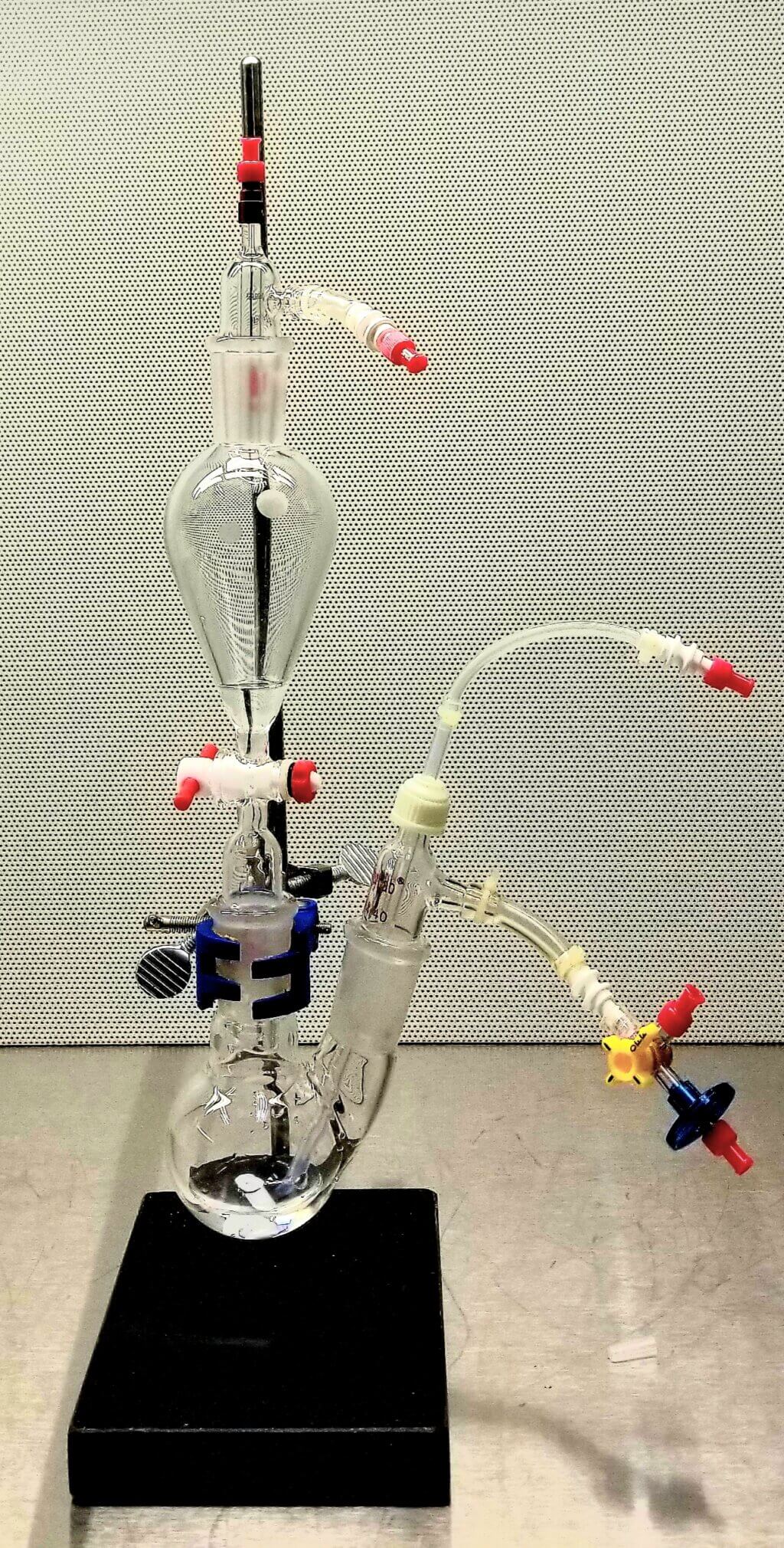
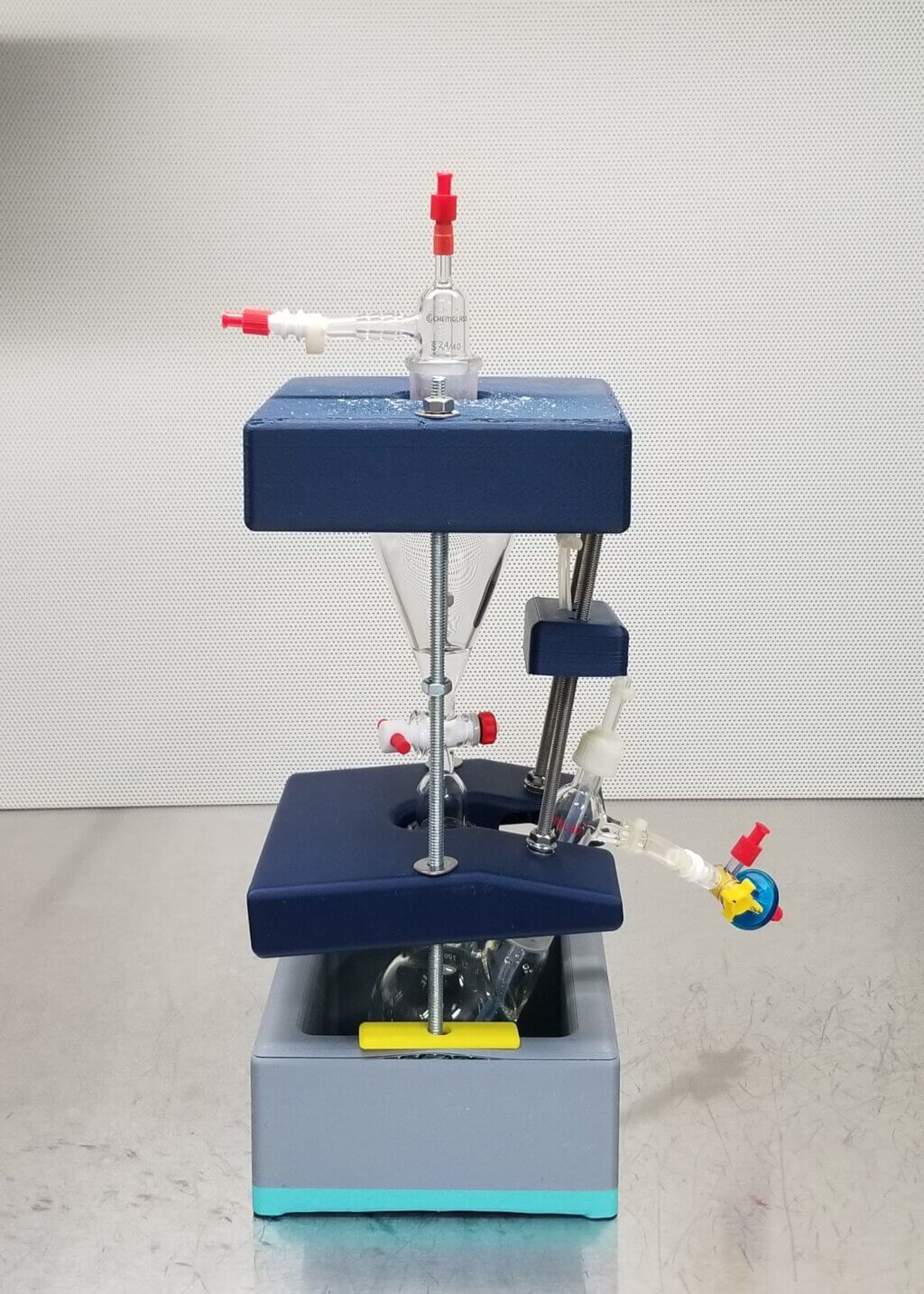
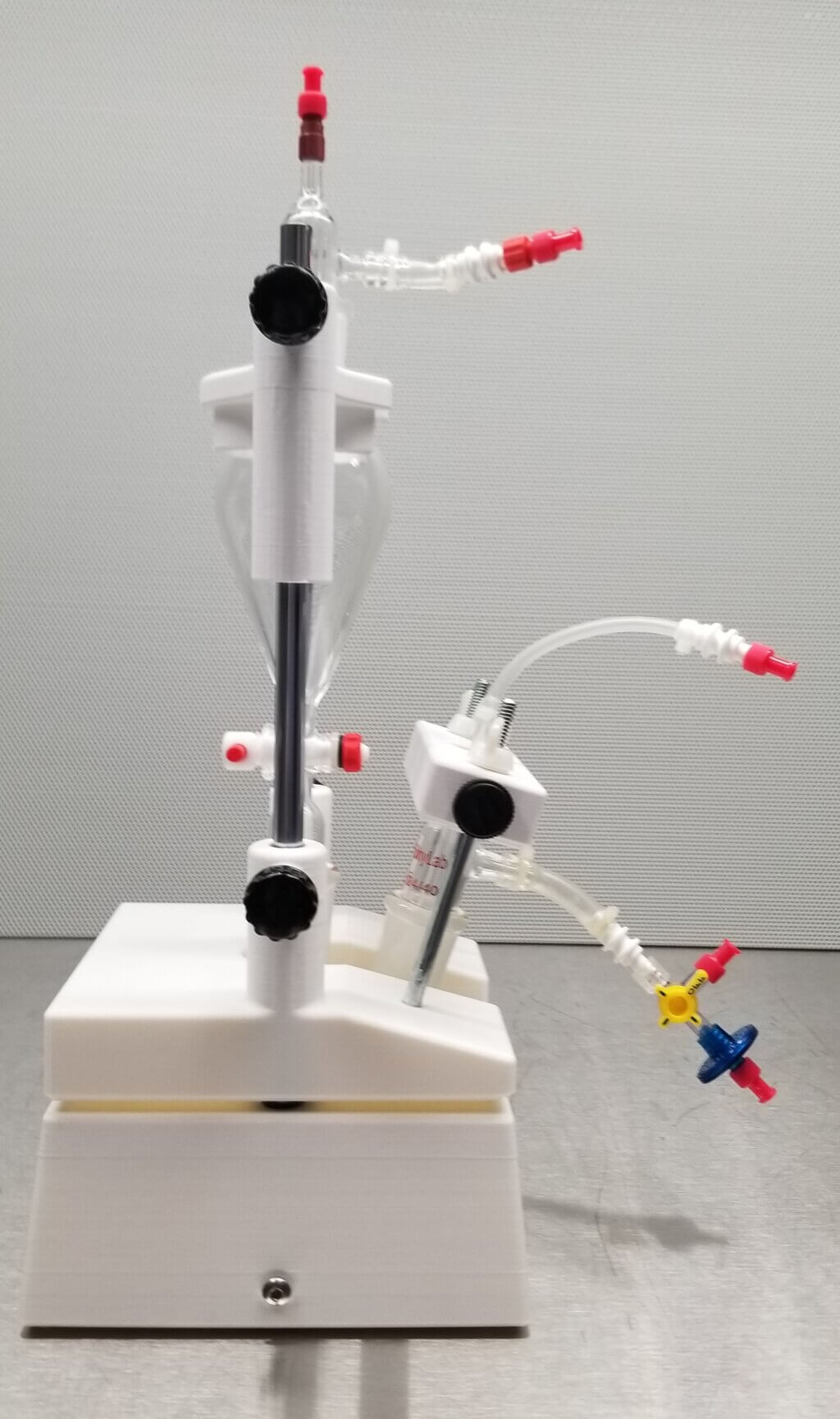
We used stands and clamps to hold the necessary equipment in our initial tests. This setup included:
- A separatory funnel: A container used to separate liquids or carry out chemical reactions, in this case, for breaking down 13C-glycerol carbonate (13C-GLC).
- A two-neck round-bottom flask: A glass container with two openings used for mixing and neutralizing chemicals.
- Adapters and fluid transfer tubing: Various small parts that help connect the equipment and move liquids between them.
This setup had several problems. It was hard to put together, increased the risk of contamination, and didn’t meet the professional standards required for clinical studies.
For the system to work correctly, it needs to handle high-pressure gas. The gas moves the solution through all the functional parts within 30 to 40 seconds and into a MedRad syringe (a special syringe used for medical injections). However, during tests, we experienced issues like parts popping off and gas leaks, which disrupt the process and increase the chance of failure in these sensitive clinical experiments.
We sought help from the Makers Lab to design and produce an apparatus for our needs. We shared an initial sketch with Makers Lab Designer Scott Drapeau. He created new sketches, modeled using computer-aided design (CAD) software and then printed and assembled the initial prototype.
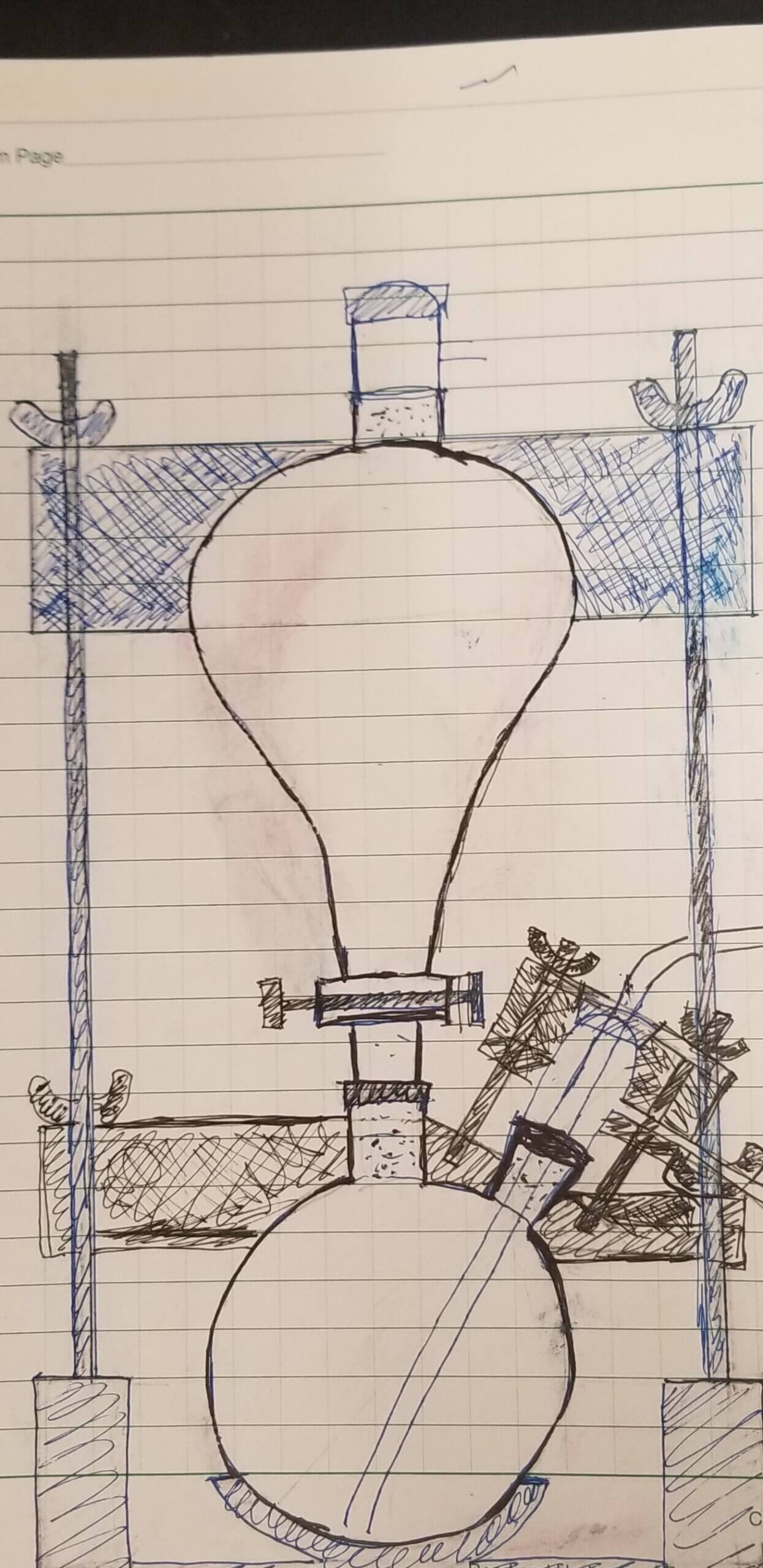
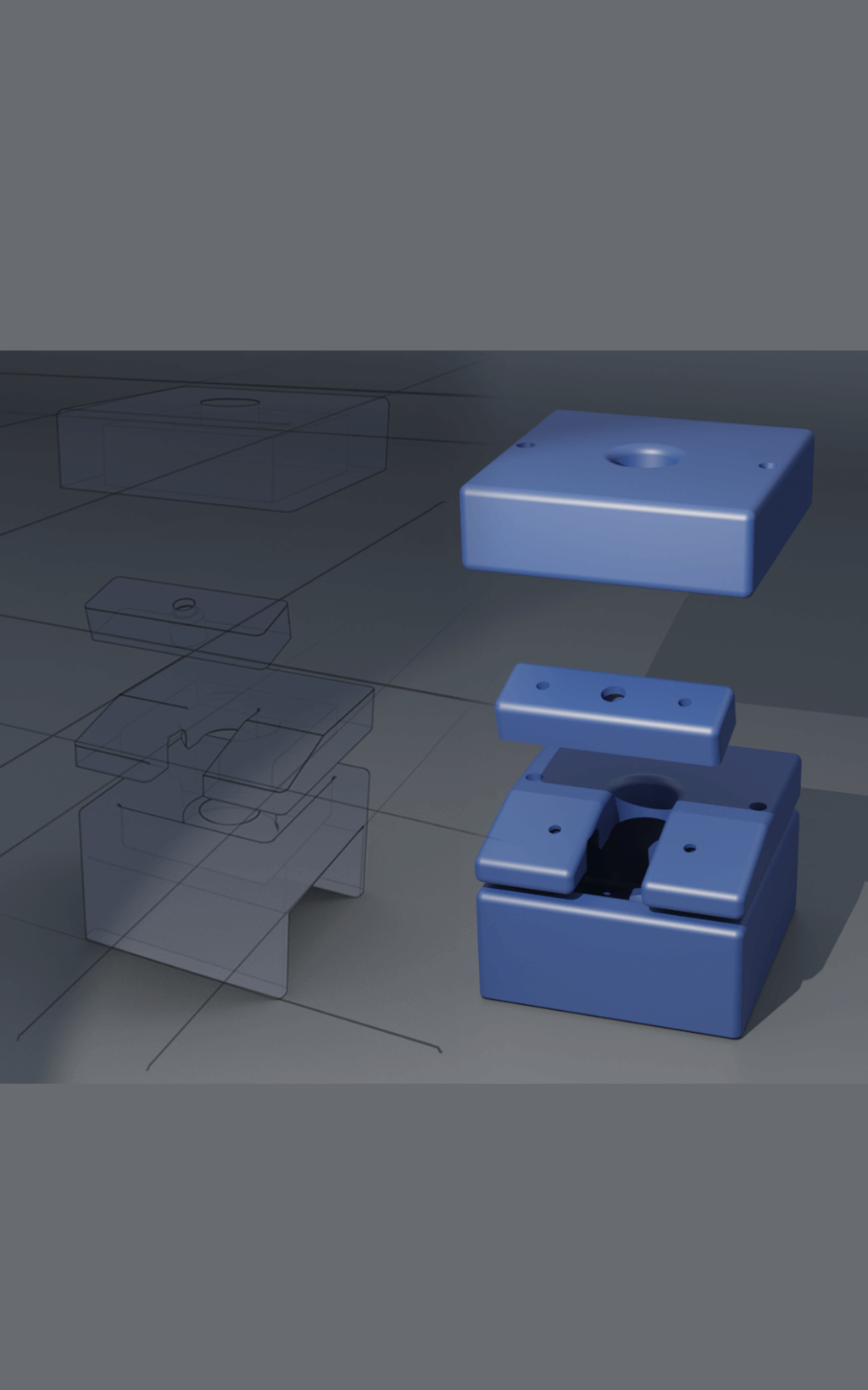
Our machine successfully addressed the earlier problems during tests, showing it could reliably produce HP 13C bicarbonate and operate safely in a clean, sterile environment. This was essential to ensure it could be used for human injections. We reported this design in our publication in the journal of STAR protocols. However, we also noticed areas for further improvement. For example, reducing the number of screws and turns needed to secure the parts could improve assembly efficiency.
Scott adjusted the design, and the updated version fully meets the standards for clinical studies, which include:
- Easy assembly
- Suitability for use in a clean room
- Excellent stability
- Excellent reliability for conducting the procedures
- A polished, professional appearance.
Also, this improved design isn’t just helpful for our research—it’s a practical solution for other hyperpolarization research centers. It allows them to adopt our tissue pH imaging method to expand their research capability.
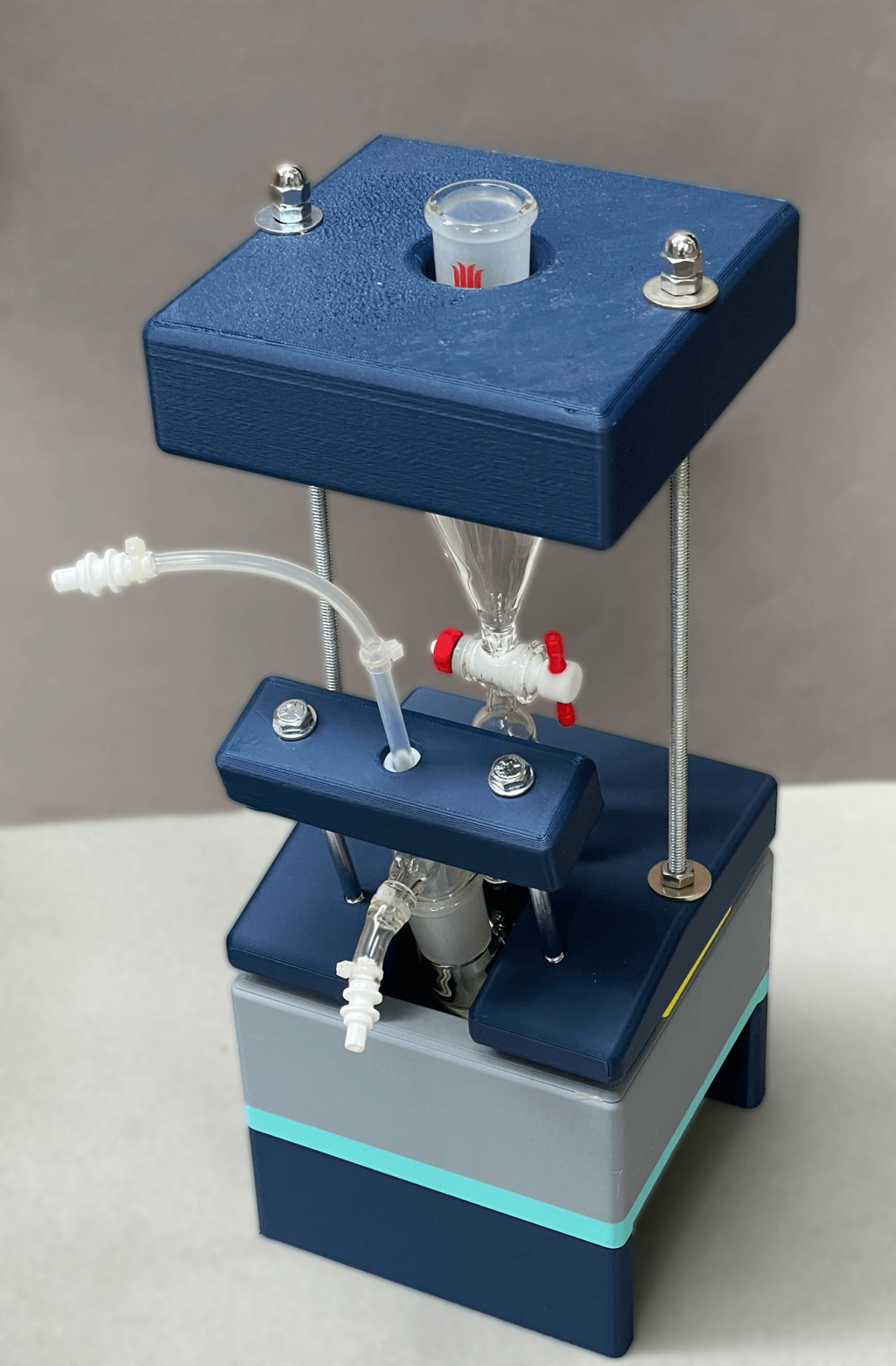
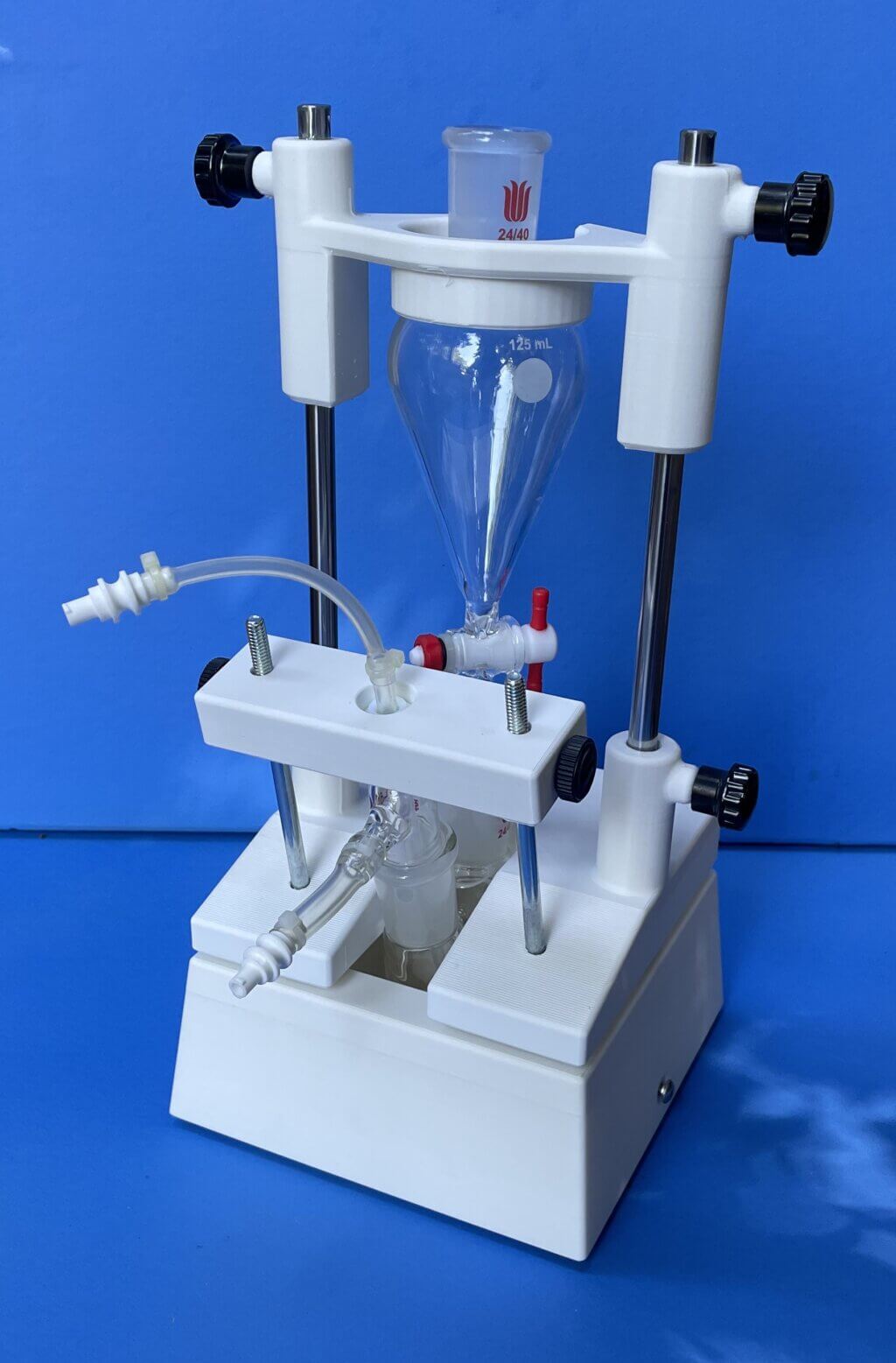
Q: What was the hardest part of the process?
The hardest part was that it took several rounds of idea exchanges and prototypes until we reached the final design. Fortunately, we have successfully obtained FDA approval for the HP 13C-bicarbonate MRI tissue pH imaging method for use in human clinical trials to assess prostate cancer status. Our goal is to disseminate this method to institutions worldwide.
Q: What was your favorite part of the process?
The rewarding part of the process was the practice-driven refinement journey. It started with sketching the initial idea on a piece of paper and, finally, refining the design for clinical application, which achieves a perfectly professional standard that facilitates global dissemination of this tumor pH imaging method.
Q: What do you want to make next?
Although we have achieved a high-quality design for the apparatus, we still have to operate the procedure manually. Our next step is to design an automated system that ensures accurate and precise control at each stage of the process, minimizing the potential for errors and ensuring compliance with standards.